SPECIAL USES, GENETICS, RESTORTATION
SPECIAL USES
Perhaps the best known product of American chestnut has nothing to do with its wood value. The flavorful nut it produces was enjoyed raw, roasted, or boiled by Native Americans and settlers, and it was an important food source for livestock and wildlife such as turkeys and squirrels. For those living in the Appalachians, chestnuts were a supplemental food and provided a source of income, 60 cents to $5 per bushel. During the late 19th century and early 20th century, chestnuts were a popular treat in large cities and could be a profitable small business venture. American chestnut wood has a narrow whitish to light brown sapwood and grayish brown heartwood that darkens with age. American chestnut is an ideal tree for many common forest products because it is rot-resistant, straight-grained, and moderately light-weight, with an average specific gravity of 0.4. It experiences moderate shrinkage and has little tendency to warp or check when drying. In the past, American chestnut was used for heavy lumber, shingles, fuelwood, telegraph and telephone poles, rails, posts, pilings, trolley and railroad ties, and even musical instruments such as pianos. Frothingham (1912) reported that chestnut was used for building, bridge and car construction, interior finish, furniture, molding, and cabinet work, and because of its uniform density, American chestnut, was ideal for annealing brass, a process requiring good temperature control. American chestnut was once the major source of tannins for leather production in the United States. With tannin content of 6 to 11 percent and numerous large logs available, American chestnut was an obvious choice for the tanning industry. Processed chestnut ended up in tanneries all over the Appalachians but also in Massachusetts, the leading leather-producing State. Dependence of the industry was such that even after most chestnuts were killed by the blight, dead trees were being harvested for tanneries.
GENETICS
American chestnut is thought to have the lowest genetic variability within the Castanea genus. The proportion of genetic diversity found among American chestnut populations is much lower than averages reported for species with a wide geographic range, species with any seed dispersal mechanism, species with similar modes of reproduction, or temperate species. Most genetic diversity appears to be within populations. Low genetic diversity could be related to the demise of the species due to ink disease and chestnut blight.
American chestnut will readily hybridize with Chinese chestnut and Japanese (Castanea crenata) chestnut, crosses of which are being developed in search of a blight resistant tree. A study by Clapper (1952) crossed American chestnut with Chinese, Japanese and Seguin (Castanea seguinii) chestnuts, as well as native chinquapin species, many of which have been more recently classified as varieties of Castanea pumila.
RESTORATION
Over the past 90 years, much research has been conducted to produce blight-resistant chestnuts using breeding techniques, biological control, and genetic engineering. Chestnuts were bred as early as the 1890s, primarily to improve nut production using hybrids of native chestnut and chinquapins with Asian and European varieties (Burnham and others 1986, Van Fleet 1914). Early breeding for blight resistance was first conducted in the 1920s by the Office of Forest Pathology, U.S. Department of Agriculture, and by Arthur Graves in his work with the Brooklyn Botanical Garden. Grave’s work was later transferred in the 1940s to the Connecticut Agriculture Experiment Station (Burnham and others 1986). Early efforts were primarily focused on developing a single cross with the desired traits that could be vegetatively propagated (Diller and Clapper 1969, Graves 1942), but the programs failed by the 1960s primarily due to an inadequate understanding of inheritance of resistance and desired form (Burnham and others 1986). Alternative breeding programs have been attempted to develop crosses that promote natural resistance among existing American chestnut populations (Griffin 2000). Researchers with the American Chestnut Cooperators Foundation cross surviving American chestnuts, and have yielded a relatively high frequency of progeny with low levels of blight resistance (Griffin 2000). Since 1986, the American Chestnut Cooperators Foundation has planted over 135,000 trees of these progenies across the native range of American chestnut. Hypovirulence of the chestnut blight has been tested as a means to control the disease (Anagnostakis 1982, Fulbright and others 1983, Jaynes and Elliston 1980). Research from Europe and the United States suggests that hypovirulent strains of the chestnut blight can weaken the pathogen (Anagnostakis 2001, Griffin and others 1983, MacDonald and Fulbright 1991). Hypovirulent strains of the blight can survive and spread for long periods in natural populations (Anagnostakis 2001). While widespread
efforts to control the blight through hypovirulence alone are probably not realistic, biological control appears to be an important part of the solution (Anagnostakis 1987, Milgroom and Cortesi 2004). Currently, the most promising option for widespread restoration of American chestnut is the development of a blight resistant chestnut variety through the backcross breeding programs of the American Chestnut Foundation and the Connecticut Agricultural Experiment Station (Anagnostakis 2002, Hebard 2005). The technique of using multiple backcrosses with the resistant parent did not begin until 1981. The breeding program includes the hybridization of American chestnut with Chinese chestnut or Japanese chestnut species that are naturally resistant to the blight. After the initial hybridization, subsequent back crossings with American chestnut progressively reduce the proportion of genetic material from the Asian parent, ultimately resulting in the third backcross hybrids (BC3F1) that are 94 percent American chestnut but retain the genes for blight resistance. After screen testing, BC3F1 hybrids that exhibit blight resistance are then intercrossed for two generations to create the BC3F3 hybrids, which retain 94 percent American chestnut genetics in progeny as well as high levels of blight resistance. The American Chestnut Foundation is currently in the testing phase of its BC3F3 seedlings through inoculations in controlled orchard settings. Early testing indicates that morphological traits of the BC3F3 hybrids are consistent with American chestnut (Diskin and others 2006), but more testing is needed to confirm that blight resistance will not break down as the trees age (Hebard 2005). The latest tests indicate the BC3F3 families are more resistant than the American parent but did not have as high of a blight resistance as Chinese chestnut. Selection is not yet complete in The American Chestnut Foundations’ BC3F2 orchards.5 The Forest Service, U.S. Department of Agriculture, also plants BC3F3 material in forest settings to test blightresistance and morphological growth characteristics (Clark and others 2011, 2012a). Early results indicate that the BC3F3 generation has height growth similar to the American parent but bud break phenology that was slightly more developed than American chestnut (Clark and others 2011). Genetic engineering of American chestnut is currently under way. Progress has been made in somatic embryogenesis to rapidly produce seedlings from multiple genotypes that have undergone genetic engineering using a wheat gene (Merkle and others 2007, Pijut and others 2011). The widespread deployment of genetically engineered trees on public lands will require addressing a number of ethical and social questions, as well as a relatively large funding base (Merkle and others 2007). Deployment of trees bred using traditional breeding efforts is now under way (Clark and others 2011, 2012a) but selections of superior families are not yet complete. It is now understood that two or three incompletely dominant genes control blight resistance (Hebard 2005, Kubisiak and others 1997), and use of genetic marker-enhanced screening would speed up traditional breeding efforts substantially (Wheeler and Sederoff 2009). Candidate genes for resistance are currently being identified (Barakat and others 2009) that can later be used in biotechnology efforts to produce a blight-resistant tree or to assist in DNA-marker assisted selection. DNA-marker assisted selection is a promising technique that could refine the selection of traits in the traditional breeding programs, resulting in more efficient breeding and reducing the time required to develop resistance (Kubisiak and others 1997, Wheeler and Sederoff 2009). Therefore, it is possible that a large quantity of seeds of blight resistant American chestnut and American chestnut varieties could be produced in the near future for the purpose of restoration. Another critical need in American chestnut restoration is to test the durability of blight resistant American chestnut and American chestnut varieties developed by the American Chestnut Foundation and the American Chestnut Cooperators Foundation, especially under various environmental stresses that are known to affect the vigor of American chestnut (Griffin and others 2006).
Park Activities
Calendar of Events
Volunteer Programs
Sky Meadows Park
Location
Geography
Habitats
Trails
Visiting Park
Crooked Run Valley
Special Projects
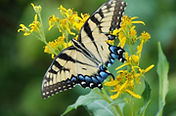